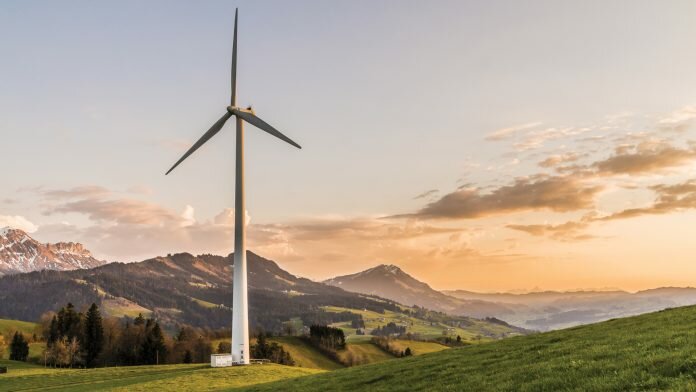
Government Europa highlights the engineering, construction and materials challenges involved in growing the European wind energy sector.
The European Commission has set a binding target of increasing the share of renewable energy in the EU’s energy mix to at least 27% of consumption by 2030, and the flourishing European wind energy sector is making an invaluable contribution to meeting that aim.
According to WindEurope, 2017 marked a record-breaking year for the European wind energy sector, with 16.8GW of additional capacity installed, up 25% on the previous year. This brings Europe’s total net installed wind power capacity up to 169GW, making wind second to only gas as the largest form of power generation capacity on the continent.
With wind energy now accounting for 11.6% of the EU’s electricity demand, it is clear that on- and offshore windfarms could play a big role in achieving the 2030 energy and climate goals, but a number of engineering, construction and materials challenges remain to be met if Europe is to unlock the full potential of wind power.
The Haliade-X
One way to boost the amount of power a wind turbine can produce is to build it bigger: increasing the size of the rotor and blades maximises the area they can cover (or ‘blade sweep’), which increases the turbine’s capacity, while taller turbines are able to exploit stronger, more consistent winds at higher altitudes, which increases their capacity factor – that is, the average amount of power a turbine generates, divided by how much it potentially could.
That’s why in March, GE Renewable Energy, a global leader in advanced technology, announced its plans to construct the world’s largest and most powerful offshore wind turbine: the Haliade-X.
The $400m (~€326m) venture, which is expected to take between three and five years to develop and deploy, will boast a 12MW direct drive generator, 220-metre rotor and 107m-long blades, allowing for a blade sweep of 38,000m2. At 260m tall it will be almost twice the height of the London Eye, raising its gross capacity factor to 63% – enabling it to produce 45% more energy than currently existing offshore turbines.
The Haliade-X is more than just an impressive feat of engineering. A single turbine alone can produce an annual 67GWh, which, according to GE Renewable Energy, is enough to power as many as 16,000 households. The company notes that a 750MW windfarm configuration could meanwhile generate enough power for one million European households.
This is good news not just for developers and energy companies but also for consumers. Being able to produce so much power from just one turbine means that windfarms can be a lot smaller, which in turn demands less capital expenditure for the balance of plant and a shorter installation cycle time, reducing the risk involved in project execution. It also simplifies operation and maintenance. The result is a lower investment and operation cost, more profitable offshore wind projects, and, ultimately, less expensive electricity.
If all goes to plan, GE Renewable Energy will supply its first nacelle for demonstration in 2019 and ship its first units in 2021.
Tackling dross
Building such commanding structures of course comes with challenges. If a wind turbine is to reliably produce power into the future – whilst staying profitable, environmentally friendly and efficient – its components must be manufactured in a way that is stable, resource-saving and at the same time cost-effective. But material defects are unavoidable in the iron casting process.
Slag inclusions such as dross have a significant effect on the load-bearing capacity of cast iron components with spheroidal graphite, which are used to make the turbine’s mainframe and rotor hubs.
The build-up of dross makes manufacturing such components extremely difficult because it requires foundries to remove the dross and ensure that only dross-free products are released for use. This is a demanding and time-consuming process which requires them to grind the raw cast part by hand.
Researchers at the Fraunhofer Institutes for Structural Durability and System Reliability LBF and Nondestructive Testing IZFP, Germany, are taking on this challenge by developing an experimentally proven dross strength classification system.
The unverDROSSen project aims to move away from the customary requirements for dross-free products and so do away with laborious post-production work.
The researchers have now succeeded at developing test methods that can be used to detect, display and characterise dross. They work by utilising mechanised ultrasound testing to display and measure the distribution of dross in a 3D format, as well as magnetic and electromagnetic methods to examine processed component surfaces.
These methods allow them to scan not just the cast underside but also the topside, which is vulnerable to dross. This could provide significant efficiency improvements across the entirety of the European wind energy sector.
“Using our non-destructive testing technology, we measured cuboids each measuring 500mm x 500mm x 200mm. We found that the dross distribution in the test pieces varied extremely. Sometimes the material defect extends across a very large surface area, and it can range in depth from a few millimetres to several centimetres,” explains Fraunhofer IZFP’s Fabian Weber. “Our results have not allowed us to derive any kind of regularity.”
While this means that components will still have to be examined individually, manufacturers should nonetheless be able to minimise necessary rework thanks to the information provided by the tests.
As the next step of the unverDROSSen project, Fraunhofer IZFP data will be used to assess structural durability. For this, the team at Fraunhofer LBF will extract fatigue specimens of 150mm x 35mm from test pieces.
“On the basis of static and cyclic test methods, we determine strength values for the different manifestations,” says project lead says Dr Christoph Bleicher, of Fraunhofer LBF.
“To do this, we place the sample in one of our test benches, pull it apart and then press each sample back together up to ten million times. Such experiments run for about ten days.”
The ultimate aim is to find out if and to what extent dross-afflicted samples weaken when put under a load such that they might fail when at maximum load, a discovery which the researchers expect will extend far beyond wind turbine manufacturing.
“In the future, we will offer a concept for reliably handling material defects in component design, manufacture and release of large cast components made of cast iron with spheroidal graphite.” Bleicher concluded that this would apply not only to the European wind energy sector, but also to the plant and mechanical engineering sector.
Materials challenges
There are several other materials challenges involved in the development of wind turbines, all of which must be solved if the true power of the European wind energy sector is to be harnessed.
Not least among these is the ability of turbines to withstand adverse weather such as extremes of temperature and exposure to UV light, as well as the impact of dust and other particles hitting them at high speed.
The development of advanced performance materials that are able to resist these conditions is now the focus of a new project by researchers at the Institute of Ceramic Technology (ITC) Agustín Escardino of Universitat Jaume I of Castellón, Spain.
AeroExtreme, which has been co-financed by the Spanish Ministry of Economy, Industry and Competitiveness, is being led by renewable energy company Siemens Gamesa and will run until the end of 2018.
It will investigate a range of passive and active solutions for the blades and nacelle of a turbine with the aim of maintaining high yields of electrical power production and high durability in extreme climatic conditions.
To this end, the ITC has developed a type of material that is able to resist erosion far better than those currently used, as well as boasting photocatalytic and antifouling coatings which further help to improve the durability of the turbine.
This should have the effect of increasing energy production efficiency while keeping maintenance and repair costs low – which will no doubt prove vital for the future of the European wind energy sector.